VII) Ozone and its Reactions
3. Determination of the OH Yield in Ozonolysis Reactions
In a previous project of the TC group, it was shown that the ozonolysis of alkenes is an important source for OH radical generation. Since OH radicals drive the chemistry of the polluted atmosphere, this finding is of general relevance for many ongoing studies in connection with air pollution. Recent studies on ethylene, butenes, and isoprene carried out by us show that the reaction mechanism is much more complicated than the Criegee mechanism, which is normally cited in connection with the ozonolysis reaction. It is a fact that a number of radicals and biradicals are involved in the gas phase ozonolysis, some of which can also lead to OH radical production. Therefore, a more complete statistical analysis of the ozonolysis considering all possible reaction channels had to be carried out to get a refined description of OH yields under atmospheric conditions.
In a first step, the kinetic quantities of the ozonolysis system were derived solving the corresponding master equation. We used a stepladder model for calculating the transition probabilities and Lennard-Jones collision numbers. The specific unimolecular rate coefficients were calculated by RRKM theory. In those cases, where a reaction channel did not involve a transition state, the specific rate constants were calculated by the statistical adiabatic channel model of Quack and Troe (J. Chem. Phys. 79, 6017, 1983). As for the molecular distribution functions we used a shifted thermal distribution in the case of exoergic bimolecular reaction, for an unimolecular decomposition of an energized precursor we assumed statistical energy partitioning between the dissociating fragments, and for unimolecular isomerization we applied a steady-state treatment. It turned out that such an approach leads to results of sufficient reliability for the discussion of the role of the ozonolysis as an OH source in the polluted atmosphere.
The project included the following steps: 1) Extension and generalization of the existing program package for carrying out the statistical analysis so that the ozonlysis of different alkenes could be analyzed for the refined and more complicated ozonolysis mechanism. - 2) Calculation of the kinetics and product distribution in the case of ethene, for which rather precise OH concentration values were available. This involved some additional calculations at the coupled cluster level to increase the reliability of results. - 3) Refinement of the PES of the butene and ispropene ozonolysis, which was described first at the DFT level and then at the CASSCF, CASCF-PT2, and CCSD(T) level of theory. For those reaction channels, where tunneling effects play a role, canonical variational transition state theory (D.G. Truhlar et al, J. Am. Chem. Soc., 101, 4534, 1979; J. Chem. Phys., 76, 1380, 1982) including the centrifugal-dominant, small curvature, semiclassical, adiabatic ground state tunneling correction (J. Am. Chem. Soc., 115, 2408, 1993) was applied.
4. Toxicity of Ozone: Ozone Reaction with Proteins
Ozone is used for the improving the quality of drinking water, the purification of wastewaters or in form of a drug to increase the oxygen content in the blood. At the same time, ozone is toxic and responsible for a number of health problems ranging from lung damage to cancer generation. Yet another aspect of the ozone chemistry has been recently reported: Singlet oxygen has been found to be a source of biologically-produced ozone: this reaction proceeds through an unusual compound dihydrogen trioxide, also known as trioxidane, (HOOOH) which is an antibody-catalyzed product of singlet oxygen and water. This compound in turn disproportionates to ozone and peroxide, providing two powerful antibacterials. The body's range of defense against all of these active oxidizing agents is hardly surprising, then, given their "deliberate" employment as antimicrobial agents in the immune response.
In this work, which is running for some years, Drs. Plesnicar and Cremer identified singlet oxygen, hydroxyl and peroxyl radicals being produced when ozone reacts with organic compounds where dihydrogen trioxide, HOOOH, plays a key role. The project is aimed at determining the formation and decomposition routes of HOOOH under different conditions in the body where both experimental and computational means are used.
The interactions of ozone with polypeptides and proteins are presently thoroughly investigated by experimentalists to determine the toxic character of ozone. In collaboration with Prof. Plesnicar (University of Lubljana, Slovenia) we investigated the intermediates of these reactions (e.g., ROOOH, HOOOH, HOOO anion or HOOO radical) using CCSD(T), and DFT.
In connection with the reactions of ozone and organic compounds we have encountered many TSs, for which DFT severely underestimates the reaction barrier because of the involvement of an odd number of electrons in the bond breaking/forming processes and an inherent large self-interaction error. For the general understanding of these situations, we developed a (Self-Interaction Corrected) SIC-DFT program that provides an improved description of the ozone TSs.
5. Identification and Characterization of the HOOO Anion
The HOOO anion (1) can adopt a triplet state (T-1) or a singlet state (S-1) where the former is 9.8 kcal/mol (ΔH(298): 10.3 kcal/mol) more stable than the latter. S-1 possesses a strong O-OOH bond with some double bond character and a weakly covalent OO-OH bond (1.80 Å) according to CCSD(T)/6-311++G(3df,3pd) calculations (the longest O-O bond ever found for a peroxide). In aqueous solution, S-1 adopts a geometry closely related to that of HOOOH (OO(O): 1.388; (O)OO(H): 1.509 Å; t(OOOH): 78.3), justifying that S-1 is considered the anion of HOOOH. Dissociation into HO anion and O2(1Σg) requires 15.4 (ΔH(298): 14.3; ΔG(298): 8.9) kcal/mol. Structure T-1 corresponds to a van der Waals complex between HO anion and O2(3Σg-) having a binding energy of 2.7 (ΔH(298): 2.1) kcal/mol. Modes of generating S-1 in aqueous solution are discussed and it is shown that S-1 represents an important intermediate in ozonation reactions.
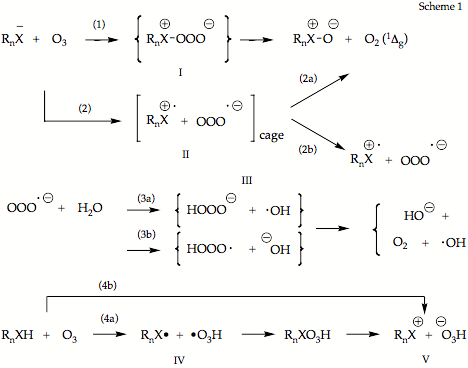
Scheme 1. Possible mechanism of ozonation reactions.
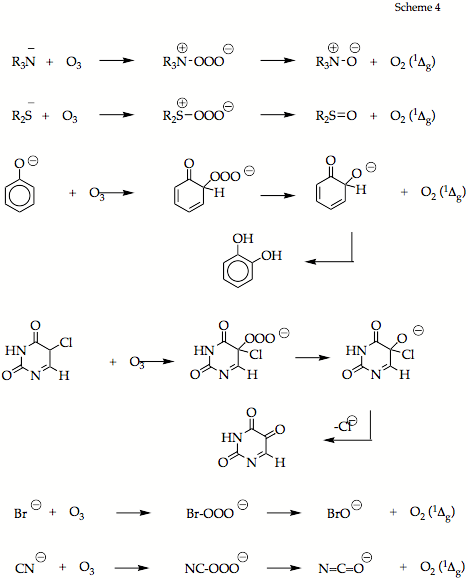
Scheme 4. Ozonation reactions proceeding via the zwitterion RnX+-OOO-.
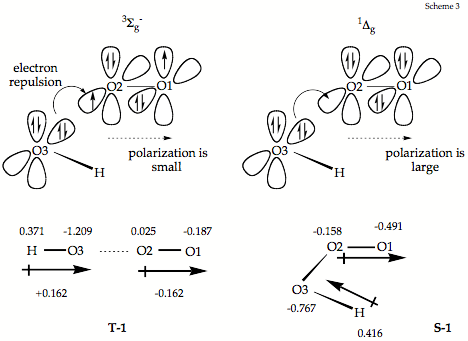
Scheme 3. Orbital diagrams and electron configuration for the interaction of the HO anion with molecular oxygen in its 3Σg- (left) or 1Δg state (right) to give T-1 and S-1.
NBO atomic charges for the CCSD(T) geometries.
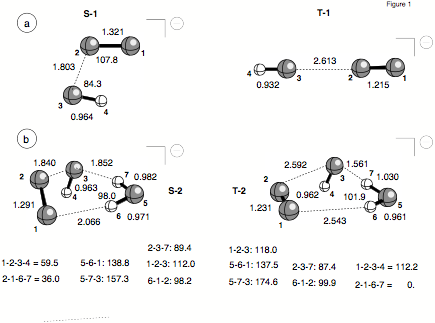
Figure 1. CCSD(T)/6-311++G(3df,3pd) geometries of 1 and B3LYP/6-311++G(3df,3pd) geometries of 2. Distances in Å, angles in degrees.
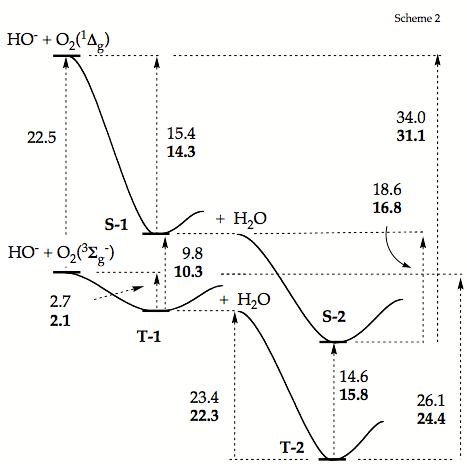
Scheme 2. Relative energies (normal print) and enthalpies ΔH(298) (bold print) of 1 and 2 according to CCSD(T)/6-311++G(3df,3pd) calculations. All values in kcal/mol.
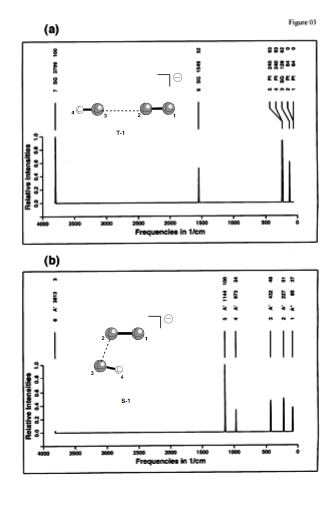
Figure 3. CCSD(T)/6-311++G(3df,3pd) infrared spectrum of a) T-1 and b) S-1.
6. Ozonation of Silanes and Germanes
Ozonation of various silanes and germanes produced the corresponding hydrotrioxides, R3SiOOOH and R3GeOOOH, which were characterized by 1H, 13C, 17O, and 29Si NMR, and by infrared spectroscopy in a two-pronged approach based on measured and calculated data. Ozone reacts with the E-H (E = Si, Ge) bond via a concerted 1,3-dipolar insertion mechanism, where, depending on the substituents and the environment (e.g. acetone-d6 solution), the H atom transfer precedes more and more E-O bond formation. The hydrotrioxides decompose in various solvents into the corresponding silanols/germanols, disiloxanes/digermoxanes, singlet oxygen (O2(1Δg)), and dihydrogen trioxide (HOOOH), where catalytic amounts of water play an important role as is indicated by quantum chemical calculations. The formation of HOOOH as a decomposition product of organometallic hydrotrioxides in acetone-d6 represents a new and convenient method for the preparation of this simple, biochemically important polyoxide. By solvent variation singlet oxygen (O2(1Δg) can be generated in high yield.
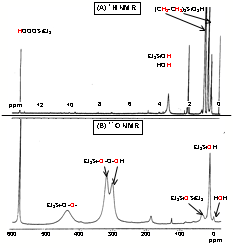
Figure 1. 1H, and (B) 17O NMR spectra of triethylsilyl hydrotrioxide (2e) at -30 °C, generated by the low-temperature ozonation of 1e in acetone-d6.
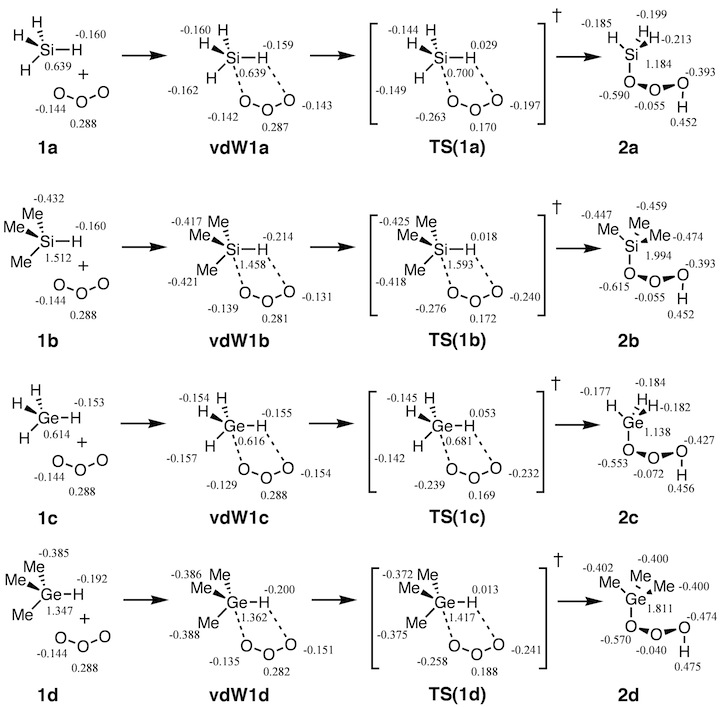
Figure 4. NBO partial charge distribution for the complexes involved in the ozonation of compounds 1a-d. Charges for compounds 1a and 1c have been calculated with the 6-311++G(3df,3pd) basis set, whereas 1b and 1d were done with the 6-31G(d,p) basis set. All calculations employed the B3LYP hybrid functional. For structures containing the methyl groups, the charges of the methyl H atoms have been summed into the carbon. For details of the calculations, see the Supporting Information.
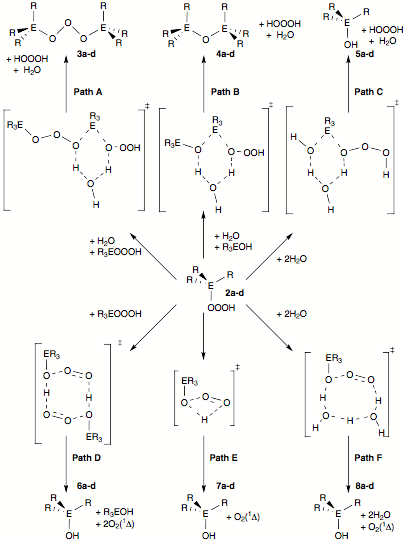
Scheme 2. Possible decomposition paths for 2a, 2b, 2c and 2d. E=Si, Ge. R=H, Me.